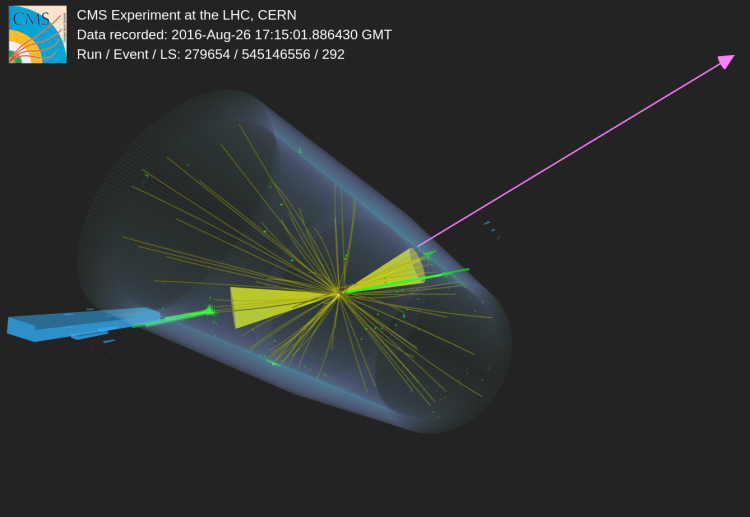
The top quark is now a full grown adult - it’s been almost 30 years since its discovery at Fermilab! - and being the heaviest brick of the Standard Model it plays a big part in the Universe. It is a bit like a celebrity in the world of particle physics because of its unique properties, quite well studied and understood in recent years. These features make it the only known particle directly interacting with almost all the other ones, and that makes the top quark play a key role in understanding the secrets of Nature, even the darkest ones.
Besides being a fascinating subject of study by itself, the top quark is a formidable tool to hunt for new forces and interactions that might be at play in the universe, and that could be still hiding in our data collected at the Large Hadron Collider. A candidate for the presence of new physics could be the W’ boson, a counterpart of the W boson, the mediator of the electroweak interaction. This new hypothesized particle could preferentially interact with heavier quarks, such as the top quark.
This analysis uses the top quark and its negatively-charged cousin, the bottom (or beauty) quark, which together make a formidable pair to go hunting for unknown particles at very high energies, where the W’ boson could hide. A top-bottom quark pair is, indeed, normally produced by the W boson, even if this process is not produced very often. A significant increase in the observed number of events could imply the existence of the new boson.
Many models addressing the unresolved puzzles of particle physics predict the existence of a new W’ boson, even more massive than the top quark (hence, heavier than an atom of gold!), possibly introducing new interactions, forbidden to the classic W boson. This game-changer particle could be produced in the proton-proton collisions of the LHC and searches have been going on at CMS since the first days, considering all the possible particles the W’ could interact with. According to Einstein’s most famous formula, high mass means plenty of potentially available energy. Imagine pouring thousands of mint candies in a soda bottle (similarly to what you can see on some youtube videos): that would result in a spectacular fast jet, and you would know for sure that you poured too many candies! Similarly, a top-bottom quark pair produced by the decay of a W’ boson would be like bullet trains traversing the CMS detector layers, expanded by the high-octane fireworks produced when the top quark also decays! This striking behavior allows us to confidently distinguish a top-bottom pair created in a W’ boson decay from all similar decay products with more conventional origins.
In practice, scientists take into account these features to disentangle the few events where the new boson is likely to be present (“the signal”) from the many others where only already known processes are present (“the backgrounds”). The better the separation between the features of the backgrounds and of the signal, the easier it is to expose the existence of the new particle. A powerful variable separating the W’ signal from the backgrounds is the total mass of the ensemble of particles hypothetically resulting from the decay of the new boson. In the measured event distribution of that variable, the signal is expected to show up as a nice peak, on the top of a continuous decreasing shape representing the background term, as sketched in Fig. 1.
Figure 1: Sketch of a falling SM background with an superimposed possible new physics signal coming from a W’ boson decaying to tb quark pair.
The analysis requires developing a clever strategy, capable of spotting the possible presence of the new massive boson with the best possible sensitivity. In other words, we need to devise an efficient method to reconstruct the W’!
The first step in the reconstruction of the W’ signal consists in selecting the physics objects recorded by CMS that are compatible with the production of a massive boson decaying to a highly-energetic top-bottom quark pair. The top quark is expected to eventually decay to another bottom quark and a muon or an electron, along with a neutrino. This process is graphically represented by the Feynman diagram shown in Fig. 2.
Figure 2: Representative Feynman diagram for the production of a W’ boson decaying in tb quark.
When these particles interact with the CMS detectors, they leave peculiar traces and energy deposits, reflecting their motion from the collision point outward. These are carefully selected and merged to obtain “reconstructed objects”. The outcome of these procedures is that the two bottom quarks emerge from the detector as “jets”, consisting of particles coming from their decays and called “b-jets” to highlight their origin. The charged lepton is more easily reconstructed with dedicated detector components. The neutrino escapes CMS without leaving any trace, but luckily produces an imbalance in the energy spread among the detected particles. This is exploited, together with the lepton and the most suitable b-jet, as a smoking gun to reconstruct the wanted top quark. The most energetic b-jet, identified as the remnants of the bottom quark directly produced from the W’, is then paired with the top quark, to get a brand new massive boson!
This strategy makes it possible to measure the mass of the W’ candidate, and eventually search for a peak in its event distribution. With dedicated statistical methods, we can assess if the measured distribution is best described when including the presence of a new particle or if the background processes are sufficient. In the second case, we can evaluate which signal configurations can be reasonably excluded. By applying this strategy to the Run2 proton-proton collision data with a center-of-mass energy of 13 TeV, collected by CMS from 2016 to 2018, we have actually found some excess in the W’ boson candidate mass distribution, at around 3.8 TeV, as shown in Fig. 3.
Figure 3: Observed and expected 95% CL upper limits on the production cross section for a right-handed W’ boson decaying to tb quarks, as functions of the W’ boson mass mW’. The W' width is assumed to be as narrow as 1% of its mass. The inner (green) band and the outer (yellow) band indicate the regions containing 68 and 95%, respectively, of the distribution of limits expected under the background-only hypothesis. The solid red curves show the theoretical expectation.
However, it is possible that this excess is actually produced by a statistical fluctuation ("by chance") of standard model backgrounds: the probability for such a lucky excess in data is about 1 out of 100, increasing to 1 out of 20 when considering that an excess of this magnitude could appear anywhere on the mass spectrum. So even if this might look exciting, it is not sufficient to declare the discovery of a new particle. Alternatively, we can assume that the W' signal is not present and exclude W’ mass values up to a certain threshold, pushing forward the previous experimental results.
The results of this study improve our knowledge about the possible existence of a new massive W’ boson, in particular for the top-bottom quark pair signature. For sure, they are very exciting and call for further investigations, soon to be made using the data currently being collected by CMS, in the Run 3 of the LHC. More studies will also be made later on, using the data to be provided by the more powerful CMS version operating during the “Hi-Lumi” phase of the LHC.
Read more about these results:
-
CMS Physics Analysis Summary "Search for W' bosons decaying to a top and a bottom quark in leptonic final states at 13 TeV"
-
@CMSExperiment on social media: facebook - twitter - instagram
- Do you like these briefings and want to get an email notification when there is a new one? Subscribe here