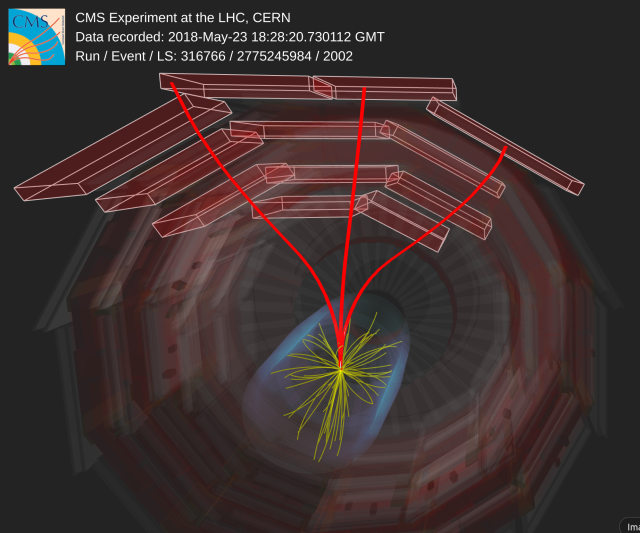
It was 1918 when the mathematician Emmy Noether published what is known as her most famous theorem, revealing the fundamental relation between the symmetries of a physical system and the laws enforcing the conservation of some of its characteristic quantities. Since then, teams of undercover detectives (called “physicists”) have used symmetries to try to unveil the secrets of Nature.
In the Standard Model of particle physics (SM), the instructions manual that physicists use, which nowadays best describes the interactions between elementary particles, there is no symmetry that guarantees the conservation of the lepton flavour. What a perfect lead for investigations! Nevertheless, it took several decades of searches for scientists to observe a process violating it.
But, wait: before going any further, let’s set the stage.
• “Flavour” is the term physicists use to distinguish between different versions of the same type of suspect (uh, particle). In our case, we’re referring to leptons, the family of particles including the electron, and its similar (but heavier) versions: the muon and the tau.
• Each of these three different-flavoured leptons has a light partner in crime, called neutrino, which doesn’t have any electric charge. However, not all neutrinos are alike: they are distinguished based on their preferred electrically charged partner: we have electron neutrinos, muon neutrinos, and tau neutrinos.
A process that violates the lepton flavour conservation is one in which the net flavour of the involved leptons changes (which is a bit suspicious, but allowed by the SM laws).
Such a process was observed for the first time in neutrino oscillations (the word physicists use to indicate the change of neutrinos’ flavour), discovered by the Super-Kamiokande and the Sudbury Neutrino Observatories, which earned the scientists T. Kajita and A.B. McDonald the Nobel Prize in Physics in 2015. No wonder we are so interested in this kind of search!
On the other hand, a violation of the lepton flavour has never been observed in processes involving charged leptons, such as the one we are looking for: the decay of a tau into 3 muons! This is potentially possible (through neutrinos oscillations), but is expected to be too rare to be observed with our experiments (Fig. 1, top). On the other hand, the scenario is quite different (Fig. 1, bottom) if we consider some extensions of our instruction’s manual (that could potentially solve several of the physicists’ headaches!). They predict a big enhancement in the probability of this process to happen, bringing this decay within the reach of our magnifying glasses.
Figure 1: How the tau→3mu process can occur (but not seen: too rare), according to the instructions contained in our usual instruction manual (top) or according to new theories (bottom).
Okay, now we can move to the next step: the investigation!
• Who: physicists of the CMS experiment
• What: the search for the tau → 3 mu decay
• When: from 2016 to 2018, when the clues (pardon, data!) were collected
• Where: at the Large Hadron Collider, at CERN, Geneva
• Why: to unveil the secrets of Nature
To maximize the result of this search, two teams of physicists have followed two different and completely independent lines of investigation:
• “Heavy Flavour (HF) channel”: the suspects, the three final muons, come from a tau lepton produced by the decay of a D or a B meson.
• “W channel”: the three final muons come from a tau lepton produced by the decay of a W boson.
Physicists from the CMS experiment were the first to follow both lines of investigations at the same time; in the past, physicists from the ATLAS and LHCb experiments carried out similar searches, but using only the W channel or only the HF channel, respectively.
The search, schematically illustrated in Fig. 2, was performed in two main steps:
1. a first skim of the suspected events was carried out by applying some selections on the quality and kinematic distributions of the muons and of the triplet they formed;
2. a more sophisticated analysis, by using a Boosted Decision Tree (investigative methods came such a long way!) was performed, to further discriminate between real tau → 3 mu events and all the others (background).
Figure 2: What physicists are looking for: evidence of the tau→3mu decay from the reconstruction of the total mass of the 3 muons (equal to the tau mass).
In the end, the results obtained from the two channels have been combined and no signal for this decay was seen (as shown in Fig. 3).
Figure 3. The measured 3-muon mass distribution, combining the analysis channels and data taking periods (black dots). The sum of the signal (background) PDF's is shown with a red (blue) line. All distributions are weighted by the inverse expected limit squared.
Therefore, the physicists placed an upper limit on the probability that this process can happen, B(tau→3mu) < 2.9 x 10-8 at a 90% confidence level, as shown in Fig. 4. The suspects have been exonerated due to lack of evidence. But the search is not over, as we continue collecting more interesting events, as the one shown in Fig. 1.
Figure 4: Upper limit on the tau→3mu branching fraction at 90% C.L., shown separately for each category and for the whole Run 2 data.
Read more about these results:
-
CMS Physics Analysis Summary "Search for the lepton flavor violating decay tau to 3mu in proton-proton collisions at sqrt(s) = 13 TeV"
-
@CMSExperiment on social media: LinkedIn - facebook - twitter - instagram
- Do you like these briefings and want to get an email notification when there is a new one? Subscribe here