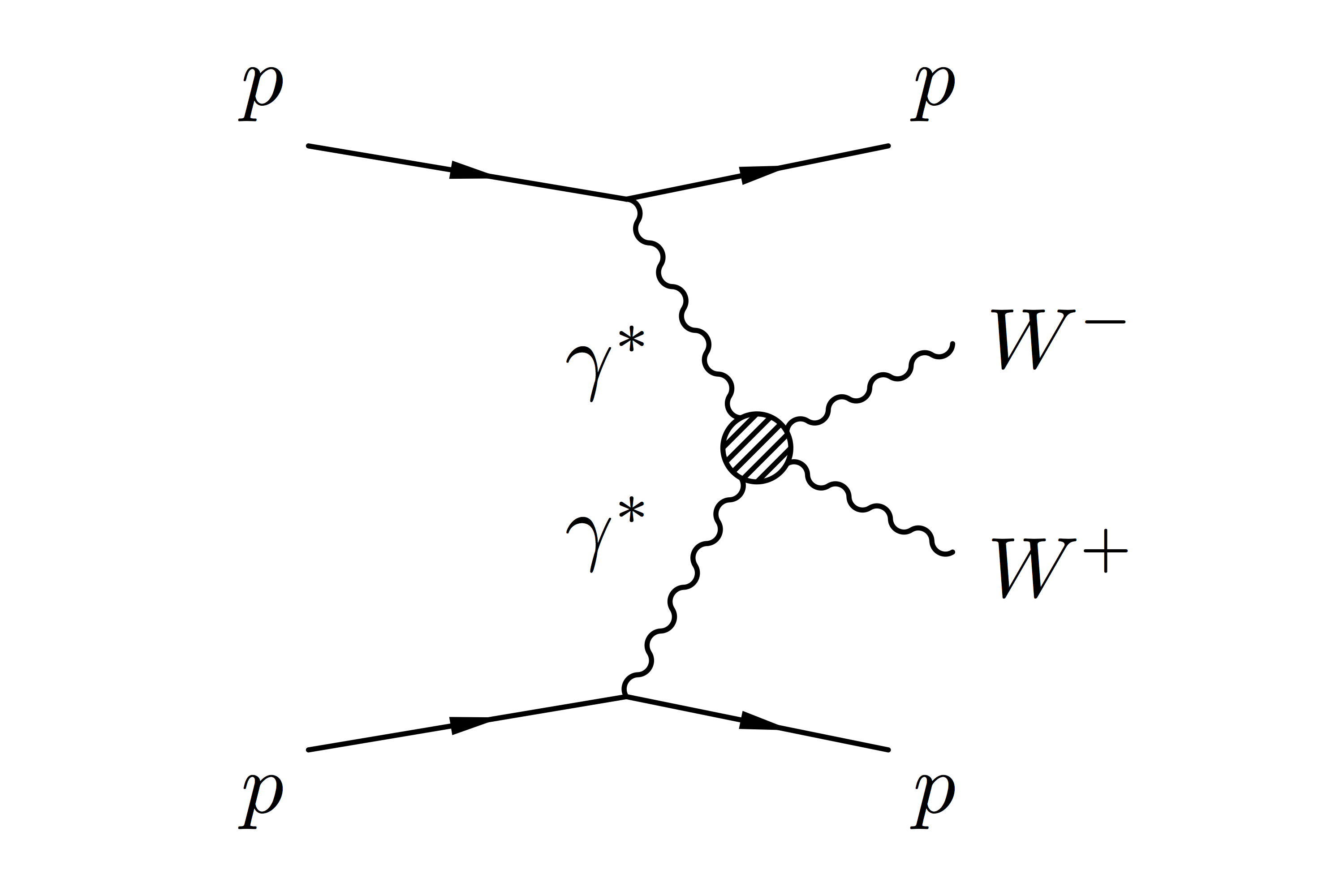
Yes, that’s correct: photon collider.
The Large Hadron Collider is known for smashing together protons. The energy from these collisions gets converted into matter, producing new particles that allow us to explore the nature of our Universe. The protons are not fired at one another individually; instead, they are circulated in bunches inside the LHC, each bunch containing some 100 billion (100,000,000,000) particles. When two bunches cross each other in the centre of CMS, a few of the protons — around 25 or so — will collide with one another. The rest of the protons continue flying through the LHC unimpeded until the next time two bunches cross.
Sometimes, something very different happens. As they fly through the LHC, the accelerating protons radiate photons, the quanta of light. If two protons going in opposite directions fly very close to one another within CMS, photons radiated from each can collide together and produce new particles, just as in proton collisions. The two parent protons remain completely intact but recoil as a result of this photon-photon interaction: they get slightly deflected from their original paths but continue circulating in the LHC. We can determine whether the photon interactions took place by identifying these deflected protons, thus effectively treating the LHC as a photon collider and adding a new probe to our toolkit for exploring fundamental physics.
This kind of proton-tagging has not been possible at the LHC so far. But a new project called the CMS-TOTEM Precision Proton Spectrometer (CTPPS) will soon enable us to study these rare collisions. The project brings together the CMS and TOTEM collaborations, which had previously worked together during the proton-lead collisions of 2013. The CTPPS will be located on either side of CMS, 200 metres away from the interaction point at the centre of the detector.
The physics case for studying photon collisions
The physics of photon collisions has been a topic of some interest for many decades. Indeed, a special meeting in 1978 discussed the prospects of such collisions at LEP, the LHC’s predecessor, which collided electrons with positrons from 1989 until 2000.
“These collisions are very clean as we’re colliding photons, which are elementary particles and not composite ones like protons,” notes Joao Varela, former Deputy Spokesperson for CMS, who is heading the CTPPS project. “It was first proposed to do this type of physics at the LHC with CMS many years ago but the project didn’t materialise then.”
One objective of the CTPPS project is to enable CMS to study quartic gauge couplings. These are interactions where the two photons annihilate upon collision to produce two W bosons: one gets four gauge bosons at the same vertex (see Feynman diagram above). “With the CTPPS, CMS can study whether the distributions and production rates of these interactions are compatible with the Standard Model or not with two orders of magnitude better sensitivity than before,” says Varela.
By locating the CTPPS at 200 metres away from the collision point, it is possible to study a mass region above 200 GeV. If there are new particles with these high masses, the CTPPS also improves CMS’s discovery potential. Varela adds, “Recently, there were two proposals in CMS and one in TOTEM to build such a spectrometer, and we put them together into a single project.”
Design and operation of the spectrometer
The CTPPS relies on objects called “Roman Pots”, which are TOTEM’s speciality. They are cylinders that allow one to move small detectors into the vacuum of the LHC in such a way that there are detectors inside the beam pipe a mere 2 mm from the beam. The tracking detectors of the CTPPS are quite small, with a surface area of 2 cm2. There will be two stations located 10 metres apart on either side of the collision point. Six planes of silicon pixels on each station will detect the track of the flying protons to give direction information. The magnetic field of the LHC’s quadrupoles will serve as the field for the CTPPS.
Once the CTPPS tags deviated protons involved in photon interactions, the CMS detector will collect the data from the collisions themselves, with information about the tagged protons embedded in the same dataset.
The Roman Pots of TOTEM are designed to operate under special LHC runs with a small number of collisions per second. The physics goals of the CTPPS will require the Roman Pots to operate during normal CMS data taking, with the LHC providing an even higher number of collisions per second from 2015 onwards. Before collecting data for physics analyses, the CMS and TOTEM teams will need to demonstrate that this operation is possible and that the CTPPS detectors can be brought very close to the beam without disrupting the beam in the process.
“One of the reasons I joined this project,” says Varela, “is to have the possibility of having detector development in a time scale that is in my lifetime. The LHC Phase 1 work is mostly done, while the Phase 2 R&D is for longer-term projects. With the CTPPS, we can make small prototypes and put them in the LHC, and start collecting data relatively quickly.”
The CMS-TOTEM Precision Proton Spectrometer will go into production in 2016.
- Log in to post comments